What can fish eyes tell us about human blindness?
My lab investigates the evolution and biological role of lens proteins called crystallins. These proteins are responsible for making the lens transparent and refracting light so that focused images fall on the retina. Amazingly, one family of crystallins, the alpha crystallins, also protect other proteins from the harmful effects of aging and stress that can lead to lens cataracts, one of the leading causes of blindness in humans. Most research on lens crystallins is performed with mammals, but we study various fish species to learn unique information about how these proteins work.
The images to the right are computer generated models for the structure of alpha A-crystallin. By comparing the versions of this protein from six fish species living at different environmental temperatures, from the Antarctic to estuaries in Florida, we were able to find three regions in this protein that may have evolved to alter its ability to protect the lens from damaged proteins. This type of comparative biology takes advantage of natural evolution to help identify changes we could make to these proteins to prevent lens cataract. We published this work in the journal PLoS One in 2012.
Keep reading below to see how we use one specific fish species, the zebrafish, to study how these alpha crystallins work.
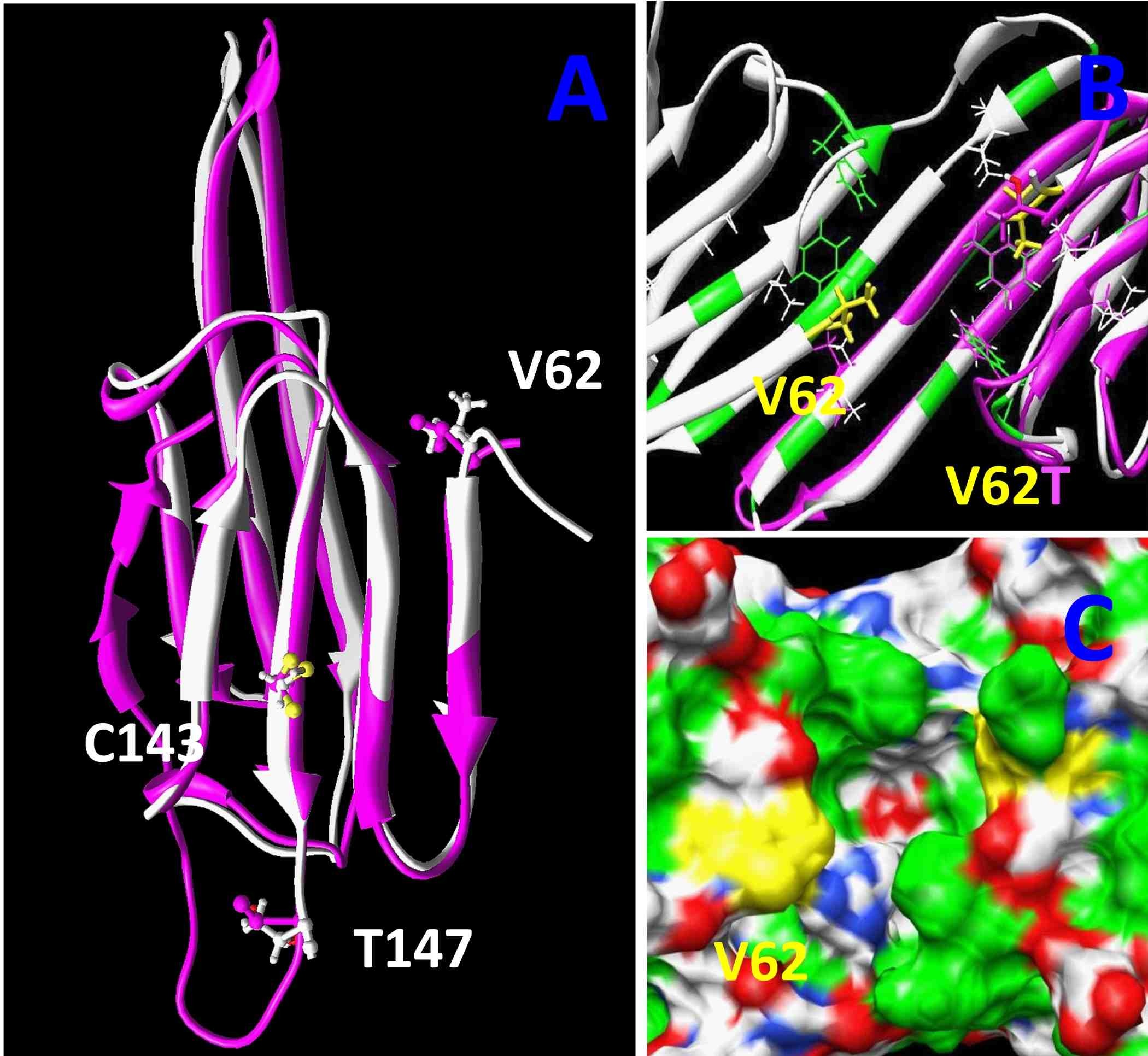
The zebrafish has become an important animal for studying development, physiology and disease because they produce a large number of small, transparent embryos that are fertilized in the surrounding water. This makes them very easy to observe in real time as they develop. We can easily visualize the lens of the eye to observe its development or presence of cataracts. The video to the left uses confocal microscopy to produce a 3-dimensional image of a cataract in a 3-day old zebrafish lens. This work was published in the journal Experimental Eye Research in 2013.
Zebrafish as a model for cataract research
The figure below from one of our recent grant proposals illustrates several other ways to image a zebrafish. The top panels compare a normal (wildtype) three day old zebrafish embryo, soon after it has hatched from its transparent egg with a mutant strain that develops an abnormal lens. Panels B and F use a type of microscopy called DIC to show that the mutant has a lens cataract (the rough area pointed to by the black arrow). The other panels show thin slices through the lens that are stained two different ways to see the nuclei within the lens cells. By three days these fish’s central lens cells lose their nuclei, helping to make the lens more transparent. In the mutant strain this process seems to stop, perhaps helping produce the cataract. The blue dots you see in the middle of the lens in panel H are nuclei stained with a chemical called DAPI.
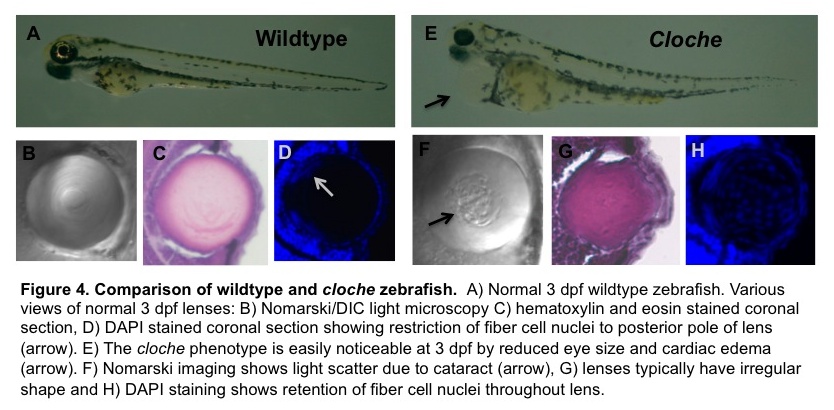
One of the reasons that the zebrafish have become such a popular species to use in biology is the growing number of ways to manipulate their genetics. By injecting various chemical into a zebrafish zygote (their one-cell stage right after fertilization) we can turn off specific genes to remove a particular protein or increase the levels of naturally occurring or even foreign proteins. These techniques give us the capacity to study the functions of lens proteins inside a living lens instead of restricting those experiments to the test tube. The video to the right shows one of these injection experiments. A red dye is add to the injection mix so that we can see where it goes.
Some additional studies in our lab

We are also using zebrafish to describe the function of gene regions called promoters that tell an organism where and when to turn on a gene. These promoters are often evolutionarily conserved so that they work across different species. The image above shows a zebrafish embryos that was injected with a mouse alpha A-crystallin promoter that is turning on the production of the jellyfish protein GFP. This allows us to visualize where the promoter is active. Because this mouse promoter works in zebrafish (and in the same way – turning on in the lens) we can use zebrafish as a fast screening tool for studying mammalian lens crystallin promoters.
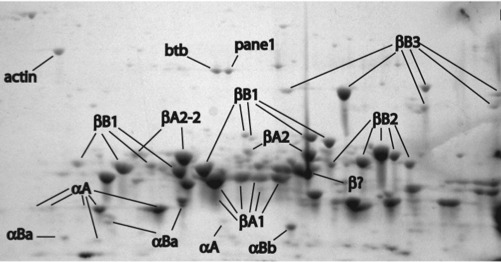
Our lab was the first to publish a description of the zebrafish lens proteome back in 2008. This study used two dimensional gel electrophoresis to separate the many proteins found in the zebrafish lens and mass spectrometry to identify each protein. Interestingly, we found that zebrafish contain a smaller amount of alpha crystallin than mammalian lenses. This is the lens protein that helps to prevent cataract during aging. Other studies of our’s showed that zebrafish alpha crystallins are comparable to the human versions in preventing the protein aggregation that can cause cataract. We continue to examine why the zebrafish may contain less alpha crystallin, although it may simply be due to very large amounts of another lens crystallin, the gammas, not allowing as much space.
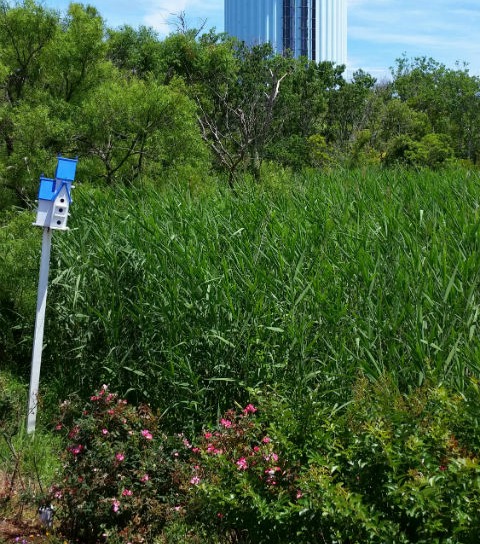
One of my research students and I had the opportunity to collaborate with colleagues from the Ashland University chemistry department on a study investigating the ability of the European grass Phragmites to invade North American habitats. My lab collected field specimens in Ohio and North Carolina (with help from my Marine Biology class) and genotyped plant tissue to confirm that they were indeed invasive, and not the native North American form. Our chemistry colleagues used HPLC and NMR techniques to show that the collected plant tissue had very low levels of gallic acid, refuting a previously published hypothesis that this toxin was the key to the invasive plant’s success.